Exocomets are raining down on Beta Pictoris – SYFY WIRE
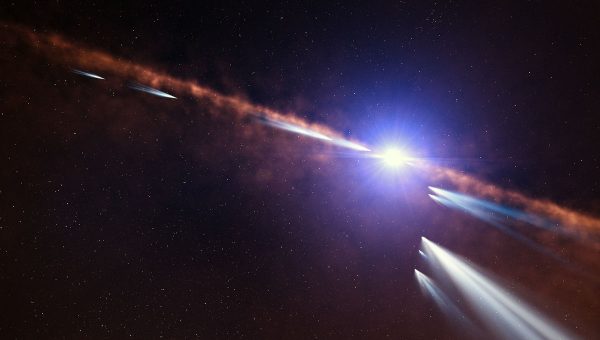
Artwork depicting comets orbiting the young star Beta Pictoris, still surrounded by a disk of material. Note that the comet tails point away from the star; that affects what we see as a comet transits the star’s face. Credit: ESO/L. Calçada
Contributed by
Sep 10, 2019
In a recent article about the nearby young star Beta Pictoris, I wrote about a second exoplanet found orbiting the star. That’s very exciting, because the system is only 65 light years away, making it easier to study than many others.
I also mentioned offhand that exocomets had been discovered there too: Literally, comets orbiting an alien star! Several have been discovered, but that highlights one in particular, because the observations of it are just so lovely.
Now mind you, comets aren’t terribly bright. Even ones in our solar system become virtually impossible to see once they’re out past Jupiter or Saturn; a comet is just a lump of ice and rock, and gets brighter near the Sun as the heat warms the surface, turning the ice into gas. That expanding coma surrounds the solid nucleus, reflecting light, and getting much brighter. But on their generally very elliptical orbits, once they return to deep space they get cold, stop outgassing, and fade away until they come back the next time (if they do).
So seeing an exocomet in a telescopic image is a long, long way off in the future. But that doesn’t mean they can’t be detected.
If a big comet is orbiting another star, and the orbit happens to be edge-on as seen from Earth, the comet will pass in front of the star’s face, blocking some of the light — that’s called a transit. Comets are small, so that’s a tiny, tiny dip… but the coma of a comet can be very large indeed. Bigger than planets. If that transits the star there can be a detectable drop in light.
This is one of the big ways we detect planets around other stars. Measure the star’s light often, and when you plot the data — what we call a light curve — you see that dip. A planet is generally spherical, so the dip in light looks like a U shape, dimming and then brightening again, symmetric.
But a comet doesn’t work that way. An exocomet transit graph doesn’t look like that. It looks like this:
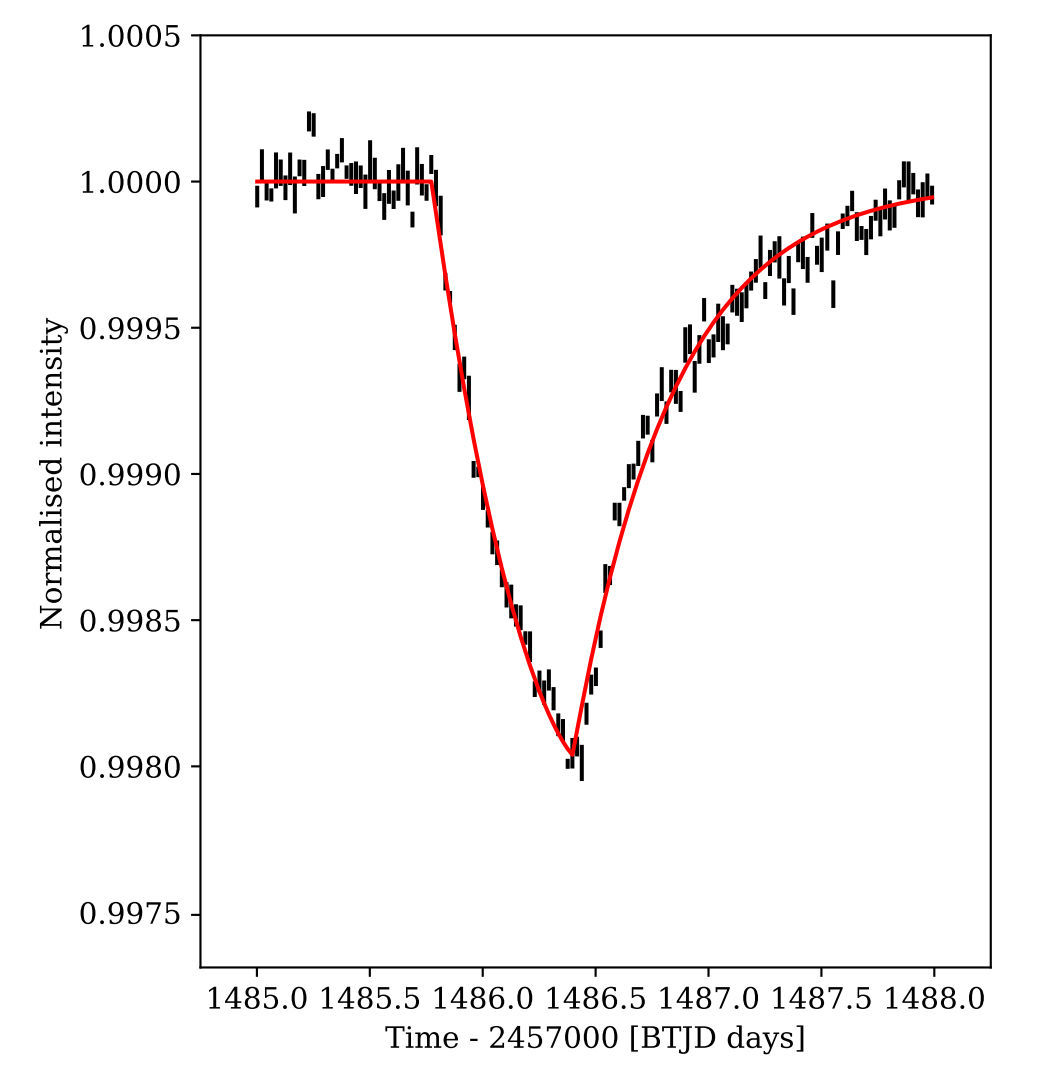
The drop in light from Beta Pictoris as a comet crossed in front of it. The black marks are observations and the red curve is a physical model fit to them. The y-axis is brightness; the comet blocked just 0.02% of the star’s light. Credit:
Oh, that makes my observational astronomer’s heart sing. That’s a great plot! Look at that fang-shaped drop in light! That’s the comet.
But how?
Think of a comet: A small solid bit surrounded by a much, much larger cloud of gas and dust. As the comet orbits the star, , literally pressure exerted on it by sunlight. The solar wind is extremely fast, so it blows the gas tail directly away from the star. But the pressure of sunlight is weak, so it exerts a smaller force. Dust from the comet blows outward, slowly, moving into an orbit farther from the star… which means it orbits more slowly. It lags behind the nucleus and coma.
So, when a comet passes in front of the star as seen from Earth, we see a fairly sharp drop in light as the coma moves across the star’s face (the left hand side of the fang). Once it passes the star’s face the light starts to rise (the right side of the fang). But it rises more slowly, because now we see the comet’s long tail crossing the star. It blocks some light, and takes longer to cross, so the rise in brightness is slower and takes longer than the dip did. This is a classic shape for a comet transit.
Not only does this indicate a comet, but the exact shape of the dip can be used to learn something about it. Using physical models of what a dip should look like as a comet passes the star, the observations can be fit mathematically to determine some of its characteristics.
It involves some complex assumptions, but in the end they find the comet’s speed was about 20 km/sec as it moved around the star at that point in its orbit, which is surprisingly slow. That’s what you’d expect for an object hundreds of millions of kilometers away from the star, whereas in reality the comet must have been much closer to the star — 1) to produce a tail at all, and b) because the farther from the star an object is the less likely we are to see a transit; even a very small tilt to its orbit makes a transit impossible.
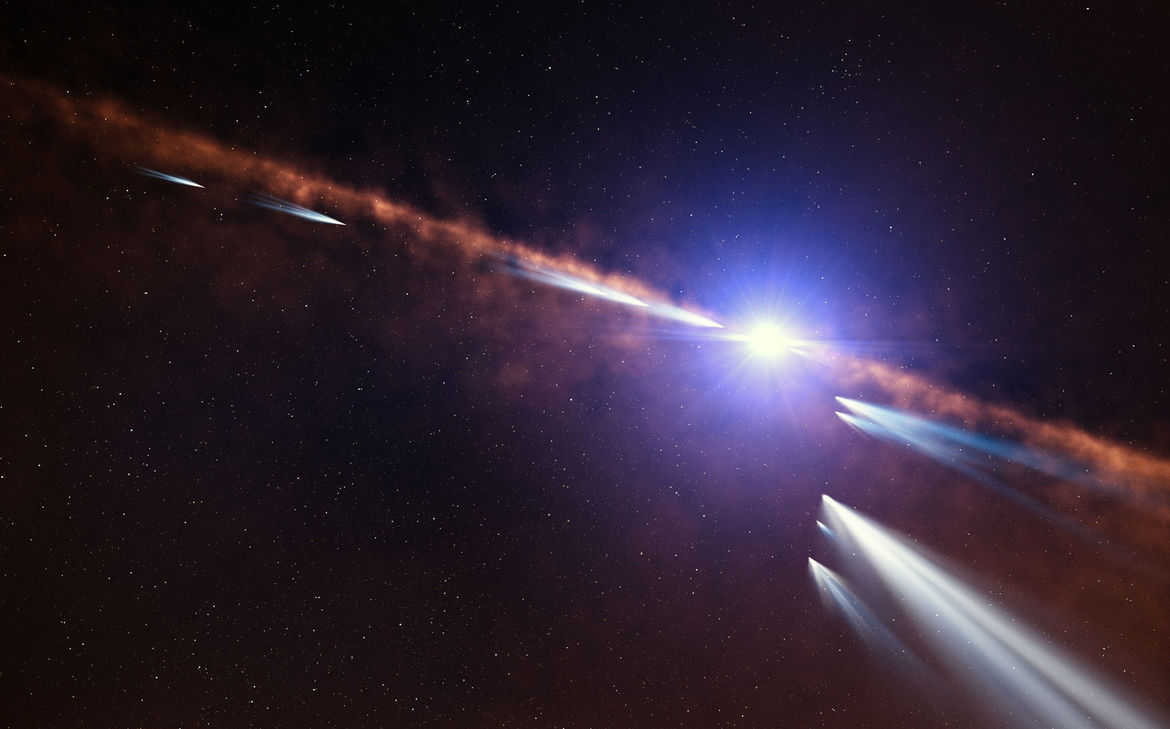
Artwork depicting comets orbiting the young star Beta Pictoris, still surrounded by a disk of material. Note that the comet tails point away from the star; that affects what we see as a comet transits the star’s face. Credit:
This made the astronomers conclude that the comet’s orbit is highly elliptical, and we see it at a steep angle, looking down the length of the orbit (if it helps you picture it, like looking down the length of a long ellipse, foreshortening it). The comet was actually moving much more rapidly, but our viewing geometry made it look slow.
Using a similar thought process, they found the tail length is probably a couple of hundred million kilometers, and is concentrated near the nucleus.
Beta Pictoris is a young star, probably only 23 million years old. For a star, that’s young! It’s still surrounded by the disk of gas and dust it formed from, and the planets still are forming from. In this disk are lots of small bodies, chunks of ice and rock, that smash together to form those planets, but there are billions more that will avoid that fate. Some will crash into the star, some will get ejected from the system, and where the planets won’t affect them, and they’ll orbit the star for billions of years.
So there’s a huge population of these objects that can pass in front of the star from our vantage point. That means there’re a lot of reasons to keep observing it. The more we do, the more we build up a database of what the conditions are like around this nearby baby star.