The enduring enigma of the cosmic cold spot – physicsworld.com
Syed Faisal ur Rahman delves into the various explanations for the strange “cold spot” in the cosmic microwave background, the ancient light of the Big Bang, that bathes the universe
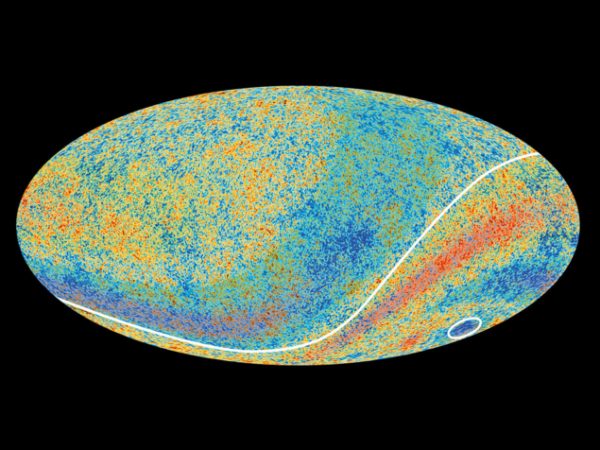
Significant events in time and space tend to leave indelible marks on the cosmos. It’s fair to say that the Big Bang – the cataclysmic event that gave rise to our universe some 14 billion years ago – has undoubtedly left its footprint on everything we observe today. The most permanent of marks, though, is its afterglow, in the form of the cosmic microwave background (CMB) – the primordial microwave radiation that fills the universe.
For the first few hundred thousand years, our new-born universe was a teeming, hot, dense plasma, made up of nuclei, electrons and photons. But once it was 380,000 years old, the universe had expanded and cooled to below 3000 K, allowing neutral atoms, including atomic hydrogen to form. Thanks to the absence of free electrons, light finally abounded the universe (from the “surface of last scattering”) and the CMB emerged. This relic radiation is therefore a perfect record of our universe during its infancy. The CMB as we detect it today has been stretched to its current microwave wavelength due to the universe’s expansion, and cooled to a temperature of just 2.7 K.
Hot and cold
At first glance, the CMB has a nearly perfect black-body spectrum (uniform temperature), and looks isotropic to scales of around 10–5 K. But at micro-kelvin scales we begin to see variations in temperature, in the form of hot and cold patches. Essentially, tiny quantum density fluctuations that occurred when the universe was just born meant that matter was not evenly distributed. Instead, some areas of the universe are more densely packed than others, giving rise to the large-scale “cosmic web” network of matter we see today. Thanks to this variation, light travelling through a densely populated region has to overcome a deeper gravitational pull, and so appears redshifted; while light passing through a less dense region will appear blueshifted.
This increase or decrease in wavelength of the CMB photons is reflected as temperature variations on the μK scale – in other words, there exists a correlation between the temperature anisotropies in the CMB and the large-scale structure of the universe. This gravitational effect, which causes the large-scale anisotropy of the CMB, is known as the Sachs–Wolfe effect, and is broken into two parts. There is the ordinary (or non-integrated) Sachs–Wolfe effect, which applies to the early universe only, and describes the gravitational redshift of light from the last scattering surface. Then there is the integrated Sachs–Wolfe (ISW) effect, which depends on the effects of changing gravitational potentials (due to matter-density variations) on CMB photons.
The primordial fluctuations in the CMB afford strong support for the theory of cosmic inflation – the extremely rapid expansion that cosmologists believe our universe underwent when it was a mere 10–35 s old. These fluctuations, along with gravitational lensing (the bending of light due to matter, as predicted by Albert Einstein), and the Sunyaev–Zel’dovich effect (the distortion of energy in the CMB photons, due to high-energy electrons in the galaxy clusters they pass through), help us to determine the relative abundance of dark matter and dark energy in the universe.
Since it was first predicted by cosmologists Ralph Alpher and Robert Herman in the late 1940s, and then experimentally detected by physicist Arno Penzias and radio-astronomer Robert Wilson in 1964 (for which the latter two shared the 1978 Nobel Prize for Physics), the CMB has provided the scientific community with swathes of information. But it has also thrown up some perplexing mysteries and anomalies, such as a significant discrepancy of the CMB as observed in the two opposite hemispheres of the sky.
Perhaps the most intriguing mystery concerns a large and unusually cold patch on the CMB, more than a billion light-years across. First observed by NASA’s Wilkinson Microwave Anisotropy Probe (WMAP) in 2004, and later confirmed by the European Space Agency’s Planck satellite, the so-called “CMB cold spot” is about 70 μK colder than the average CMB temperature, and appears in the southern celestial hemisphere.
While it’s possible that the cold spot could have originated from the primordial density fluctuations that created the rest of the CMB’s temperature anisotropies, it’s unlikely. Those anisotropies have a Gaussian distribution, which allows for small variations (on the scale of 18 μK), but not large ones. But in some places, the cold spot is nearly 150 μK colder than the mean CMB temperature, far in excess of that expected from a Gaussian distribution. Also, the radius of the cold spot subtends about 5°, whereas the largest fluctuations of the primordial CMB temperature occur on angular scales of about 1°, making it even more unnatural.
It should come as no surprise then that researchers the world over have posited a number of theories regarding the CMB cold spot, and many studies are being done to uncover its origins. Some early explanations suggested that the cold spot could merely be foreground contamination (in the form of galactic dust and synchrotron radiation) from within the Milky Way, or the result of unusual celestial objects. But observations by the NRAO VLA Sky Survey (NVSS), the 2 Micron All-Sky Survey (2MASS) and the Sloan Digital Sky Survey (SDSS), along with detailed images from the Hubble Space Telescope, all showed no such objects.
1 It all started with a Big Bang
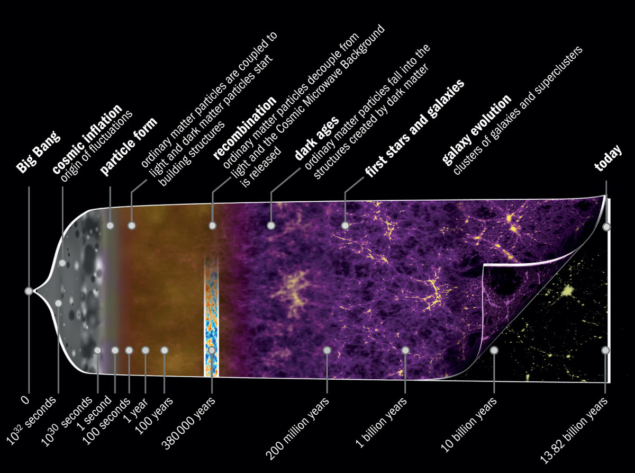
An illustrated history of our universe, going back 14 billion years to the Big Bang, showing the main stages of its evolution.
Veiled void?
One promising explanation for the phenomenon is that there exists a vast cosmic “super-void” between us and the cold spot, thanks to the large-scale structure of the universe. While galaxies still exist within voids, their density of matter is much less (one-tenth of the average) than in other regions of the universe. On the flip side, the universe also contains superclusters – huge regions with many more galaxies than normal.
As it turns out, both super-voids and superclusters have a significant impact on CMB photons due to a variation of the integrated Sachs-Wolfe effect, known as the late-time ISW effect. Essentially, CMB photons passing through superclusters – gravitational “valleys” – gain potential energy as they roll into the valley, and so heat up. All things being equal, this energy should be used up by the photon, as it climbs back out of the valley when leaving the supercluster. But all things aren’t equal because dark energy, which causes the accelerated expansion of our universe, comes into play.
In the time it takes the photon to travel across the supercluster, dark energy stretches the valley, somewhat flattening it, such that the exiting photon does not need all of the extra potential energy to climb back out. The CMB light therefore holds on to some of the gained heat. In a similar way, super-voids are considered gravitational “hills”, and CMB photons lose more energy while entering a void and climbing uphill than they regain as they roll downhill while leaving the (now somewhat bigger) void. This loss in energy chills the photons. The ISW effect could therefore be a promising explanation for the CMB cold spot, should a super-void exist in the direction of the spot.
The late-time ISW effect could be a promising explanation for the CMB cold spot, should a super-void exist in the direction of the spot
Hide and seek
Indeed, in 2007 a study by Lawrence Rudnick and colleagues from the University of Minnesota claimed to observe a super-void in the region, thanks to a significant dip in extragalactic brightness and number-counts of radio sources in the region, as seen in the NVSS radio catalogue. But a 2010 follow-up study, by Kendrick Smith of the University of Cambridge and Dragan Huterer from the University of Michigan, found no statistically significant evidence for the aforementioned dips in NVSS maps, and so discounted the existence of a super-void. A 2014 study, led by Seshadri Nadathur of the University of Helsinki, does not debate the existence of a large void in the area, but claims that the ISW impact on the CMB from the proposed super-void is nowhere near enough to explain the cold spot.
The Helsinki researchers further claim that in order to see the necessary photon-cooling via the ISW, the super-void would have to be so massive and so empty that its very existence would contravene the standard model of cosmology for our universe, known as ΛCDM. This model suggests that the universe is governed by the competing forces of dark matter (which accounts for 26.8% of the universe’s mass/energy) and dark energy (68.3%). The gravitational tug of dark matter works against the accelerated expanding force of dark energy. Nadathur’s work finds that any structure capable of explaining the cold spot would be an anomaly itself.
On the other hand, also in 2014, a team of astronomers led by István Szapudi of the Institute for Astronomy at the University of Hawaii claimed to find a rare super-void, almost 1.8 billion light-years across, in the region. At the time, Szapudi described it as “the largest individual structure ever identified by humanity”. Using optical observations from Hawaii’s Pan-STARRS1 (PS1) telescope, combined with infrared data from NASA’s Wide Field Survey Explorer (WISE) 2MASS catalogue, the team surveyed relatively nearby galaxies that lie within the cold spot’s boundaries, and detected a super-void. According to Szapudi’s team, the void is a mere three billion light-years away from us, which may be why it was not detected in previous searches that focused on the distant, early universe. Despite its discovery, the researchers found that even this large super-void does not fully account for the CMB cold spot’s temperature drop, as the cooling ISW effect would be a maximum of 20 μK.
Temperature test
In an attempt to test Szapudi’s discovery, a 2017 study led by Ruari Mackenzie of the University of Durham used spectroscopic data from the 2dF-VST ATLAS Cold Spot Redshift (2CSz) survey at the Anglo-Australian Telescope in New South Wales to study the redshift of galaxies in the line of sight to the cold spot. As a control for their work, the researchers also made the same measurements along a different line of sight. Mackenzie’s team found three voids out to a distance of three billion light-years, and a possible fourth void beyond that. Although no individual void was as large as Szapudi’s super-void, the four combined showed an ISW cooling effect of 31 μK – still not enough to explain the cold spot.
Unfortunately, the researchers found a similar density of voids along the control line too, which led them to believe that voids are not the answer. Instead, they conclude that the cold spot may indeed have somehow originated in primordial density fluctuations. As it stands, the presence of Szapudi’s super-void is difficult to explain in the standard ΛCDM, while simulations show that a random, non-Gaussian quantum fluctuation in the CMB has a 1 in 50 chance of birthing the cold spot.
Unusual structures?
Another explanation for the CMB cold spot could be the unusual motion of galaxies in the region, due to extreme gravitational effects. This could be similar to the effects observed due to phenomena such as the “Great Attractor” – an apparent gravitational anomaly at the centre of the local Laniakea Supercluster (within which our Milky Way galaxy is located) caused by an enormous concentration of mass – and the related “Dipole Repeller”, which is a centre of effective gravitational repulsion in the large-scale flow of galaxies in our Local Group, caused by the likely presence of a large super-void. Both of these have their own imprints on the CMB dipole, but so far no such unusual structures have been observed in the CMB cold spot area or the surrounding regions.
A November 2019 study by Qi Guo and colleagues at the Chinese Academy of Sciences reported the existence of 19 dwarf galaxies that are dark-matter deficient, which is surprising because these small galaxies are usually dominated by dark matter. Of these galaxies, 14 are isolated, and not satellite galaxies to large ones like ours, which means that their lack of dark matter was not the result of some interaction with larger galaxies or other dwarf galaxies. These low-dark-matter dwarf galaxies may affect the results of other ISW studies, all of which are impacted by dark matter. It will be interesting to see if a significant number of galaxies in the CMB cold spot region exhibit such behaviour.
Perhaps the answer to the CMB cold spot lies within the theory of inflation itself, instead of beyond it. It might be that during the inflationary epoch in our universe’s infancy, a local patch of the universe underwent a longer period of inflation, which resulted in the formation of a cold spot in that region. That’s the solution put forth in 2016 by Yi Wang, of the Hong Kong University of Science and Technology, and Yin-Zhe Ma, of South Africa’s University of KwaZulu-Natal, in which they proposed a “feature-scattering” inflationary mechanism that predicted localized cold spots (and no hot ones). However, questions will arise about the validity of such theories if we look at other phenomena, including the effect of such anomalies on density perturbations, and the evolution of the stars and galaxies in that region.
Several solutions
As researchers attempt to uncover which solution is the right one for the cold spot, it’s important to make sure that each explanation is considered in the larger context of other cosmological observations such as observations of type Ia supernovae, baryonic acoustic oscillations (BAO), and the overall CMB observations themselves. It has also recently been suggested that a more accurate measurement of the Hubble constant could be made using the gravitational waves from the merger of heavy objects like neutron stars. These observations, with some variations in the values of the measured cosmological parameters, support ΛCDM and so any workable solution must agree with them.
However, alternatives to the Standard Model will also play an important part in exploring anomalies such as the cold spot. For example, in a 2019 study Eleonora Di Valentino of the University of Manchester, and colleagues, carefully analysed the Planck 2018 CMB data, and their finding challenges our usual ΛCDM assumption of a flat universe. Their results point to a “closed universe”, which contradicts our current assumptions, and deviates from our usual understanding of inflation theory. While their results were inconclusive (just over 3σ), this issue undoubtedly needs to be further investigated.
2 Compare and contrast
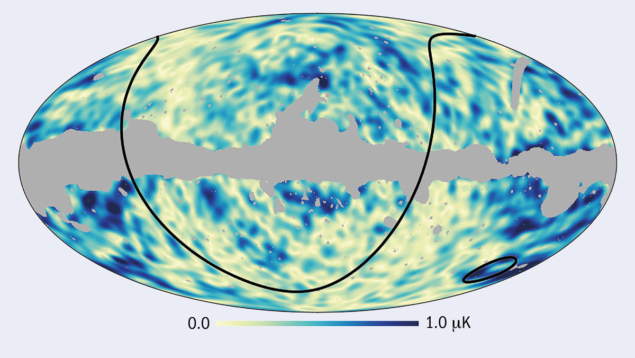
The Plank satellite observes the CMB in detail, at large angular scales (≥ 5°). The top map depicts the CMB’s temperature fluctuations, and the bottom map shows the polarization amplitude fluctuations. While the temperature map clearly shows the cold spot, the anomaly was not detected, at least with any statistical significance, in the polarization map. The lack of statistically significant anomalies in the polarization map does not rule out the potential relevance of those seen in the temperature map, but makes it more challenging to understand the origin of this puzzling feature.
A more exotic explanation?
Thanks to the lack of a clear-cut explanation for the CMB cold spot, a more unusual and out-there possibility has been suggested – that the cold spot might be evidence of a “collision” between our universe and a parallel universe. This falls under the multiverse theory, according to which our universe is one of many that occasionally collide or interact with one other. Parallel universes could have interacted with ours thanks to quantum entanglement between the universes before they were separated by cosmic inflation, and interactions would leave a mark on the CMB. But again, extraordinary claims such as this one require extraordinary evidence, and should be consistent with other cosmological observations.
In this case, such a collision between universes should produce an identifiable polarization signal in the cold spot, as suggested by Tom Shanks of the Centre for Extragalactic Astronomy at Durham University in 2017. Incidentally, the latest results from the Plank team in 2019 involved a further analysis of the polarization of the CMB radiation (which is almost completely independent from its temperature profile), to further probe the nature of anomalies like the cold spot. Planck’s multi-frequency data are designed to eliminate foreground sources of microwave emission, including gas and dust in our galaxy. Despite careful analysis, the Plank team saw no significant traces of anomalies in the polarization maps (figure 2). According to the team, these latest results neither confirm nor deny the nature of anomalies like the cold spot, leaving the door open to many possibilities.
Improving our understanding of the cosmological parameters will provide better possible explanations of unusual phenomena like the cold spot. For this, we require new data from highly sensitive telescopes such as the MeerKAT array and upcoming facilities like the Giant Magellan Telescope and the Square Kilometre Array. We also need to develop a better understanding of the nature of dark energy and how it affects the evolution of our universe, to get deeper understanding of the working of the ISW effect.
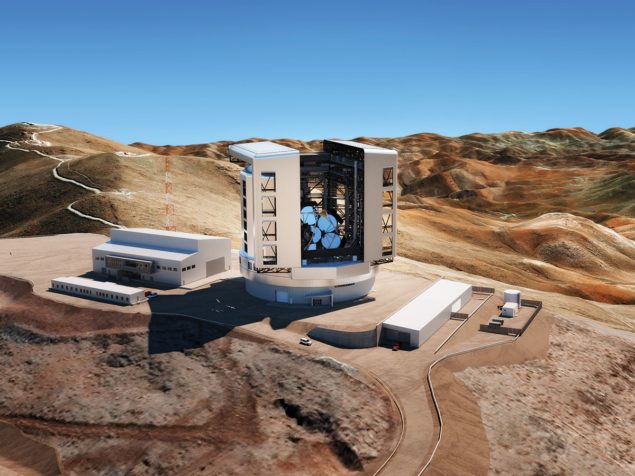
As it stands, our current understanding of the CMB cold spot doesn’t lead us to any clear conclusions. Fully understanding it either requires much better observations, or a revision of our understanding of the universe. Hopefully, future observations from more advanced ground- and space-based telescopes can guide us towards a better explanation of this enigmatic scientific phenomenon.